Can Ocean Energy Power Carbon Removal?
Study Finds Offshore Energy Could Help the World Reach Critical Carbon Removal Goals (And a Bubbly, Soda-Like Method Might Be Our Best Bet)

The Caribbean has a problem, and it stinks.
Atop the Caribbean Sea’s famously pristine waters floats a 5,000-mile-wide heap of rust-colored, brambly seaweed. When that seaweed, a form of sargassum, clumps up on beaches and decomposes, it emits hydrogen sulfide gas (also known as swamp gas), which smells like rotten eggs and, in high doses, can be toxic. For obvious reasons, this seaweed swarm is a huge problem for the Caribbean’s tourism industry and residents—and potentially for Florida, where the heap is headed next.
But this stinky seaweed could also be part of a solution.
“If you sink that seaweed into the deep sea, you can potentially avoid those issues,” James Niffenegger said. “And with seaweed sinking, the deeper you go, the longer you can store the carbon dioxide it absorbed from the air and water.”
Niffenegger, a researcher at the National Renewable Energy Laboratory (NREL), is an author of a new study funded by the U.S. Department of Energy’s Water Power Technologies Office. The study examined various methods to capture carbon dioxide from the air or ocean and permanently sequester or store it—or, better yet, do both simultaneously.
These techniques—called marine carbon capture, marine carbon sequestration, and marine carbon dioxide removal—are almost as diverse as marine wildlife: Some involve farming or sinking seaweed, others inject captured carbon into deep-sea rocks, and some capitalize on clever chemistry to remove carbon directly from the ocean. But almost all are relatively new and untested technologies, and their costs, environmental impacts, and potential efficacy are still largely unstudied.
Until now.
For their study, Niffenegger and his colleagues—David Greene, Robert Thresher, and Michael Lawson—analyzed the benefits and drawbacks of each of the most promising marine carbon management techniques. But they also looked at how the country—and the world—could power these carbon-snatchers, especially those that operate in the remote ocean, far from any power grid.
The ocean, the team found, could be a valuable partner. Offshore energy technologies, including wind turbines and marine energy devices—which generate energy from ocean waves, currents, tides, and other watery power sources—could help meet global carbon removal goals. And they could do that with the energy available in U.S. waters alone.
“This is not a cure-all,” Niffenegger said, meaning carbon removal alone cannot halt climate change.
Still, it is one remedy we can no longer do without.
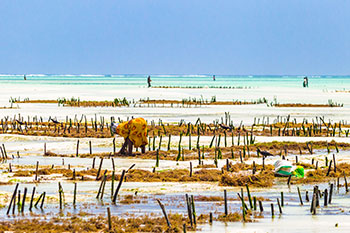
A Sinking Ship: Why Carbon Removal Is No Longer Optional
Like on-land carbon capture technologies, which can extract carbon from our air, marine carbon capture harvests the molecules from seawater or the air above. Carbon causes problems for both: Excess carbon dioxide gas in the atmosphere creates a kind of blanket around our world, trapping more heat as more carbon crams in. And even though the ocean absorbs a lot of that airborne carbon, those waters can only trap so much. Plus, too much carbon causes ocean acidification—a steady increase in seawater acidity—which puts marine ecosystems and wildlife at risk. Today, the ocean is basic, with a pH similar to baking soda.
“Basically, we’re in a sinking ship now. Our boat is taking on water and we’ve got to plug up the holes,” Niffenegger said. “But even after we plug up the holes, we’ve got to bail the water out. And if we take too long to do that, there might still be too much water in there for us to avoid the most significant impacts.”
According to the Intergovernmental Panel on Climate Change, carbon dioxide removal is now essential to keep global warming to 1.5 degrees Celsius. If we cross that marker, today’s climate change crises—superstorms, wildfires, floods, extreme droughts, lethal heatwaves, crop devastation, and more—will only get worse. To avoid those catastrophes (and their economic and human costs), the world needs to limit warming to 1.5 degrees Celsius by 2100. And to do that, we need to remove about 3 to 7 billion tons of carbon from our atmosphere per year by 2050. (For context, humans emitted about 40 billion tons of carbon dioxide in 2022 just by burning fossil fuels).
But the growing carbon dioxide removal industry will, like any other industry, require energy to pull that carbon out of our atmosphere and ocean. “The amount of energy that’s going to be needed would essentially require us to double the energy generation capability of the current grid in the United States,” Niffenegger said. That is if the country were acting alone, but even so, the U.S. grid accounts for about 16% of the world’s total energy generation.
Burning fossil fuels to power carbon removal would be a Sisyphean effort: pointless and arduous. But there is another option, one that could, according to Niffenegger’s new study, power enough carbon removal to limit global warming to that critical 1.5 degrees Celsius.
There is enough offshore wind and marine energy above and within U.S. waters to power the removal of 10 billion tons of carbon dioxide per year, according to Niffenegger.
That is huge. But not every technology designed to remove or capture and sequester marine carbon dioxide can get us to that number. And each, the research team found, comes with trade-offs. One might cost less but capture less carbon. Some can only operate in remote, hard-to-reach locations. Others consume high amounts of energy or come with hefty environmental risks.
“I want to try to be fair to all the different methods,” Niffenegger said. “They all have potential.”
Seaweed, Acid, and Chalk: The Many Types of Marine Carbon Dioxide Removal
Most marine carbon capture, sequestration, and removal technologies are still in the early stages of development. But some, like offshore seaweed and algae farming, have been around for more than a century.
“We’ve been doing that for a very long time,” Niffenegger said. “Not in the United States, but in Asia. It’s very simple.”
Like trees, sea vegetation, including seaweed and microalgae, absorb carbon dioxide from the air and store it in their slimy cells. Three of the marine carbon management methods the NREL team analyzed rely on this biological hunger for carbon: seaweed farming, microalgae farming, and artificial upwelling. Artificial upwelling, in which nutrients are pumped up from the deep ocean to fertilize shallower waters, causes algae to bloom along the surface.
But these methods mainly just capture carbon; they do not necessarily store it for long periods of time.
That is where the sinking comes in: Pushing all that captured carbon to the ocean floor by sinking seaweed could store it for a few hundred years—unless it gets eaten on the way down. If fish, krill, and turtles gobble up the seaweed feast, how long does that carbon remain stored in a stomach or scaly body? Hard to say.
And a sunken smorgasbord comes with a deadlier risk.
A sudden influx of food in the deep ocean could attract crowds of wildlife, which consume the plants but also oxygen. Because sunlight doesn’t reach most of the ocean floor, plants can’t grow, and nothing breathes new oxygen into the area. “That can lead to hypoxia, low oxygen zones, and kill off more life,” Niffenegger said.
So, while seaweed and algae farming, seaweed sinking, and artificial upwelling are all relatively low-cost options for carbon dioxide capture and removal, they come with trade-offs. All require lots of energy and could cause severe environmental damage if they capture the amount of carbon needed to fight climate change.
But other, non-biological methods could reach those lofty numbers with fewer environmental risks.
These so-called electrochemical methods rely on the ocean’s chemistry rather than its vegetation. For the study, Niffenegger and team explored three types of electrochemical carbon capture and removal techniques, each of which use electricity and some specialized membranes—or fine filters—to separate seawater into acidic or basic solutions. All three techniques can capture carbon. They can also produce hydrogen (which can be sold as fuel by itself or combined with the carbon dioxide to make synthetic fuel) and chlorine gas (which is toxic on its own but can be used to manufacture certain products, like disinfectants).
The first electrochemical method, called acid stripping carbon dioxide, converts ocean-based carbon into a gas, which bubbles out, like a freshly cracked-open can of soda. But those bubbles do not just float away into the atmosphere; they can be caught and sold to make fuel.
This technique also produces a basic liquid as a byproduct—a happy one. Because the initial chemical reaction turns the seawater acidic, this basic byproduct can be poured back into the water to balance its pH before it is returned to the ocean.

The ocean is one of our greatest climate allies, absorbing heat and about 30% of global carbon emissions. But these waters have already sucked up too much of our excess; it is getting too warm and too acidic. It cannot keep up.
Unless, that is, we can get rid of some of that built-up acid—which is exactly what the second electrochemical method does. Called base addition, the technique injects a basic solution directly into seawater to reduce its acidity. The third method, carbonate formation, turns the ocean’s carbon into carbonate, a chalky substance that can then be removed and used in building construction.
Unlike acid stripping, these second two techniques permanently remove carbon rather than just capture it. But they also produce a lot of acid.
“You could sell some of it, but I don’t know if the global economy can handle billions of tons of acid,” Niffenegger said. “It will likely need to be safely disposed of.”
These chemistry-based technologies are more expensive but could be our best bet, according to the study. They could remove up to 10 billion tons of carbon dioxide per year—potentially enough to bail out our sinking boat.
And yet, like seaweed farming, carbon capture techniques, including the soda-like electrochemical method, also need a safe way to store their captured carbon dioxide for hundreds or even thousands of years. Niffenegger and the team examined carbon sequestration techniques, too. Companies could, for example, submerge liquid carbon dioxide, which is denser than ocean water, deep in the ocean. These liquid carbon lakes could last for up to a thousand years, but they can also trap—and kill—wildlife unlucky enough to be beneath the lake when it forms. To avoid that environmental risk, companies could instead inject carbon directly into an inert seabed or rock formation, where it could remain for hundreds of millions of years.
“Injecting carbon dioxide into the seabed and mineral reservoirs requires more research,” Niffenegger said. “But there’s interest in seeing what each of these can do. Can we safely do this in the ocean or not?”
A Perfect Pair: Ocean Energy and Marine Carbon Dioxide Removal
Whether onshore or offshore, carbon removal technologies will require substantial amounts of energy to achieve global targets. But ocean-based carbon removal comes with a few extra benefits, like vast open space and few concerns about technological eyesores. Onshore, carbon capture and sequestration companies might need to divert energy away from the U.S. power grid to power their technology, but offshore, there is plenty of renewable energy flowing through ocean waters and winds. And much of that energy simply crashes ashore, unused.

“There is a massive amount of energy that exists offshore that the grid likely won’t even be able to use since it’s so far from shore,” Niffenegger said. “But you have to make sure that you’re doing everything safely.”
Offshore carbon capture, sequestration, and removal companies will need sensors to monitor potential environmental impacts as well as how much carbon their technology captures, stores, or removes. But plunging sensors down to the seafloor, embedding them next to deep-sea rock formations, or even tethering the tech to a buoyant, wave-rocked seaweed farm are not easy tasks.
At least one solution is clear: Predictable, reliable marine energy—especially wave energy—could help power all those sensors and at least some of these offshore operations. In some cases, it already does: Some offshore microalgae farms use wave energy to mix their vegetation, which encourages growth. Offshore wind energy packs an even bigger punch and could power the bulk of marine carbon removal efforts, and when those winds do not blow, steady marine energy can help fill energy gaps.
Marine energy technologies are still in the early stages of development; companies are working to hone their designs to make them durable enough to withstand the ocean and cost-effective enough to be commercially successful. But Niffenegger sees an opportunity for the marine energy and marine carbon dioxide removal industries to codevelop their budding technologies.
“This is a preliminary investigation, but it shows a lot of promise,” Niffenegger said. “But, like I said, this is preliminary.”
Even if marine energy is relatively consistent, waves can still ebb and flow. Researchers must investigate whether these dips could impact carbon removal efforts. But Niffenegger’s study shows that marine energy and marine carbon removal could be a mutually beneficial partnership.
“I’m just trying to get people interested in trying this,” Niffenegger said. “There’s a lot of potential opportunities for collaborating between these two fields.”
Check out the study, Mission Analysis for Marine Renewable Energy To Provide Power for Marine Carbon Dioxide Removal, to learn more about marine carbon dioxide removal. And subscribe to the NREL water power newsletter, The Current, to make sure you do not miss a water power update.
Feb. 1, 2024 | By Caitlin McDermott-Murphy