Arctic Changes: Where my polar bear go?
Convergence science, integrating diverse fields, is crucial for addressing complex issues like those in the Arctic due to climate change and industrialization. Experts advocate for this approach, demonstrating its utility in analyzing Arctic stressors and systems through a holistic lens, exemplified by studies on the Yamal Peninsula.
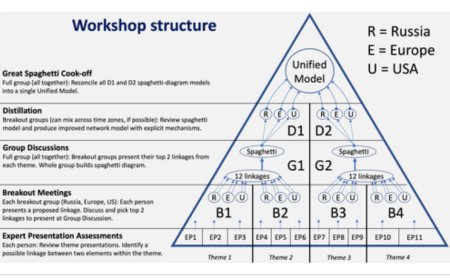
This paper represents a synthesis of conceptual analyses, case study analyses, and practical thoughts on the application of convergence science in Arctic change studies. During a virtual workshop in 2020, a diverse, multi-national team of authors consisting of social scientists, engineers, earth system scientists, and ecologists came together to formulate broad, scientifically, and societally important questions on how the Arctic system in the Yamal Peninsula of Western Siberia responds to pressures of rapidly changing climate and increasing industrialization. The team “engineered” a novel approach for expert (representing a disciplinary domain) and non-expert (representatives of other disciplines) communication and at the workshop conclusion developed several convergence science questions of high appeal. Three of such questions are presented in this manuscript to illustrate how the search and identification of appropriate mechanistic linkages are critical to the development of system-level understanding of stressor impact propagation. The need to understand underlying disciplinary and cross-disciplinary mechanisms connecting Arctic system elements is viewed to be an inherent part of the convergence science approach. Through pursuit of such understanding, the approach naturally leads to other novel emerging questions, thereby stimulating further application of the process of integrative thinking.
Abstract
Science, engineering, and society increasingly require integrative thinking about emerging problems in complex systems, a notion referred to as convergence science. Due to the concurrent pressures of two main stressors—rapid climate change and industrialization, Arctic research demands such a paradigm of scientific inquiry. This perspective represents a synthesis of a vision for its application in Arctic system studies, developed by a group of disciplinary experts consisting of social and earth system scientists, ecologists, and engineers. Our objective is to demonstrate how convergence research questions can be developed via a holistic view of system interactions that are then parsed into material links and concrete inquiries of disciplinary and interdisciplinary nature. We illustrate the application of the convergence science paradigm to several forms of Arctic stressors using the Yamal Peninsula of the Russian Arctic as a representative natural laboratory with a biogeographic gradient from the forest-tundra ecotone to the high Arctic.
Key Points
-
Arctic research demands convergence science as essential method to understand impacts from novel stressors
-
An integrative approach is developed by a diverse team to formulate questions that cannot be fully addressed within disciplinary studies
-
A convergence science analysis is illustrated for three questions applicable to Yamal, Russian Arctic, a microcosm of the changing Arctic
Plain Language Summary
This paper represents a synthesis of conceptual analyses, case study analyses, and practical thoughts on the application of convergence science in Arctic change studies. During a virtual workshop in 2020, a diverse, multi-national team of authors consisting of social scientists, engineers, earth system scientists, and ecologists came together to formulate broad, scientifically, and societally important questions on how the Arctic system in the Yamal Peninsula of Western Siberia responds to pressures of rapidly changing climate and increasing industrialization. The team “engineered” a novel approach for expert (representing a disciplinary domain) and non-expert (representatives of other disciplines) communication and at the workshop conclusion developed several convergence science questions of high appeal. Three of such questions are presented in this manuscript to illustrate how the search and identification of appropriate mechanistic linkages are critical to the development of system-level understanding of stressor impact propagation. The need to understand underlying disciplinary and cross-disciplinary mechanisms connecting Arctic system elements is viewed to be an inherent part of the convergence science approach. Through pursuit of such understanding, the approach naturally leads to other novel emerging questions, thereby stimulating further application of the process of integrative thinking.
1 Introduction
Given the drastic, rapid, and concurrent changes in the high latitudes and their impacts on global processes and peoples of the North, the Arctic represents a complex system that warrants urgent integration of research efforts. The necessity for integrative approaches addressing cumulative and compound effects of multiple drivers of changes has been highlighted in recent reports (AMAP, 2021; Arctic Council, 2016) emphasizing the need for the Arctic research community to step away from the relative comfort of disciplinary silos and move toward the development of holistic system views and novel paradigms.
Arctic research demands convergence science.
How can convergence science be construed? In contrast to the plain meaning of its etymon (the Latin convergere) to “incline together,” convergence research as a novel type of scientific endeavor encompasses not just passive integration of knowledge or a cascade of boundary conditions from one disciplinary group to another. On the contrary, it calls for the identification of fruitful research areas of opportunity to foster the emergence of new views, scientific principles, and even disciplines—the process in which diverse participants operate with a common language and reference points (Sharp & Langer, 2011; Thompson et al., 2023). In theory, a true application of the convergence science approach, individual disciplines and traditional concepts intersect, fuse, and cross-pollinate to gain novel insights and to understand emergent complexity, while accelerating solutions to big, complex problems (Stokols et al., 2008).
How can convergence science approach be implemented, given the various cognitive and social barriers (Wagner et al., 2011) associated with the number of and relative separation among the disciplines? In practice, convergence science enhances research through interdisciplinary teams of scientists and stakeholders working together to push the scope of scientific inquiry beyond the typical boundaries of their respective fields, to foster mutual learning and novel collaborations, and develop a transdisciplinary language and knowledge consolidation to solve specific problems and respond to demands from society. For the purposes of this paper, we use the definition of transdisciplinary research offered by Rosenfield (1992) (“researchers work jointly using shared conceptual framework drawing together disciplinary-specific theories, concepts, and approaches to address common problem”) and view it as a required element of convergence science (Thompson et al., 2023) that focuses on big, complex socially-relevant problems. The implementation is not without challenges and tensions: identifying disciplines needed to address complex system-level problems, selecting compelling questions that will emerge as research foci, and integration and application of diverse methodologies require “engineering of communication” among experts and distillation of integrative perspectives.
The central objective of this paper is to illustrate finely grained objectives and processes of convergence science, moving away from the “generalist,” broad contemplation level, to the application of the concept to specific Arctic stressors and mechanisms they imply. We seek to showcase how this approach allows the identification of linkages critical to the development of system-level understanding of stressor impact propagation. In the process of developing that understanding, we uncover knowledge gaps falling within the scope of both interdisciplinary and discipline-specific research. Concurrently, this paper also aims to demonstrate how a diverse group of author-scientists, who were trained largely within the niche of their single discipline, can through integrative thinking advance questions and understandings in ways that cannot be achieved with studies in their “host” disciplines alone.
To provide an intuitive application of the concept, we use the Yamal peninsula in the Western Siberia (Russia) as a case study region for this synthesis, as evidence indicates increasingly intertwined processes caused by multiple stressors on the abiotic, biotic, and socioeconomic systems over the past four decades. Combining responses to these multiple drivers of change, Yamal is a vivid illustration of the need for convergent scientific understanding of Arctic change. Three representative convergence science threads are developed in this paper.
2 Facets of Convergence Science of Arctic Change
The Arctic is subject to several types of novel stressors that evince multiple levels of interaction with its environment and inhabitants. Here, we focus on two specific stressors that likely incorporate the larger fraction of unknowns and concerns across scientific and stakeholder groups and therefore constitute immediate research needs: climate change and industrialization. We explore how convergence science can trace the effects of stressor impacts across systems and may support genuine synthesis and shared understanding across disciplines.
Decadal changes in temperature of the near-surface atmosphere in the Arctic have profound implications for the loss of snow and ice and thus their feedback to regional and global climate (Hinzman et al., 2013). Surface temperature changes are related to the air energy content (Graversen et al., 2008), which is an approximation of air heat content (i.e., the sum of enthalpy and latent heat, which are the respective functions of air temperature and humidity) (Pielke et al., 2004). Due to profound effects of the latter on the various dynamics, we use heat content as the metric of “environmental conditions.” We consider this climactic driver a primary forcing factor of change in the abiotic, biotic, and human systems.
An increase in near-surface heat can be conveyed via weather, event-scale impacts due to heat waves characterized by peak temperature and humidity as well as duration above a threshold. Gradual increase in the warming and duration of the above-freezing period leads to climate-scale changes, such as the timing of season onset and termination, their average heat content and duration. We consider changes for both types as external, that is, without accounting for how the Arctic land-surface will feedback to them. Even with this simplified view, we can distinguish two concepts. First (a), there can be temporal persistence of processes triggered by both pulse-scale and climate-scale changes (e.g., a brief heat wave may trigger an over-a-threshold behavior with long-term consequences; likewise, gradual changes in annual seasonalities may cause incremental but continuous changes in the Arctic system under consideration). Second (b), the impacts of heat content changes are often overlapping, and may lead to multiple feed-forward and feedback loops in the systems of impact, among which we target those that have longer-term implications. Arguably, mechanistic, process-level understanding of linkages generated in (a) and (b) constitute the core of Arctic climate change impacts that are of interest to science and society (Hinzman et al., 2013; Meier et al., 2014; Overland et al., 2016; Walsh et al., 2020). We consider the complexity of relevant pathways by using both temporal scales associated with an increase in the atmospheric heat content and consider questions that require a convergence science approach.
The second stressor about which we are concerned is industrial development. Globally, industrialization is defined as a period of social and economic change during which people's means of gaining subsistence shifts to minimize human drudgery and improve predictability of resource availability via systematization and simplification of processes, an extensive division of labor, substitution of mechanical for human energy, and replacement of small, localized, and uncertain sources of supply by large, networked and controllable ones (Shimkin, 1952). Industrialization is a complex phenomenon with many regional and temporal elements that result in particular histories and complex constellations of identities and socio-political groupings. Industrialization is also an accelerator of acculturation that has affected every society across the globe, as people in less industrialized societies borrow or adapt to features of more industrialized cultures. Industrial societies have all experienced dramatic increases in the per capita production of food, services and goods through the mechanization of manufacturing and agriculture. Industrialization depends on the social systems that provide labor (Robertson, 1991). A social system consists of individual human beings interacting with one another within certain continuing associations and institutions.
Russian industrialization of the Arctic is characterized by large-scale operations associated with the exploitation of non-renewable resources, and the construction of cities built around extraction cites and transportation networks (Zamyatina, 2023). This was accompanied by a large-scale influx of workforce who settled first temporarily, then permanently in such cities (Bolotova & Stammler, 2010). Alongside the development of non-renewable resources, indigenous peoples across Siberia, the Russian Arctic, and the Far East were incorporated into the state agricultural system, first through trading cooperatives linked to state procurement agencies, and then in the 1930s into collective farms (kolkhoz), and 1960s state farms (sovkhoz and gospromkhoz) for exploitation of renewable resources (mainly reindeer, fish, and fur) (Ziker, 2002). Oil and gas resource development, intensifying after the breakup of the USSR, is more distributed and reliant on the construction of linear infrastructures, such as pipelines, and exploitation of shift-worker regimes, rather than construction of industrial cities (Saxinger, 2016). Although recent expansion of oil/gas and mining associated infrastructure in the arctic has mostly occurred in Russia, there are also significant developments in the US and Canada (Bartsch et al., 2021).
2.1 Climate Change
2.2 Industrialization
3 Arctic Microcosm—Yamal, Western Siberia
We regard the Yamal Peninsula as a natural laboratory with Pan-Arctic explanatory relevance, because of the concentration of a large variety of pertinent components in the earth and social-ecological systems. These characteristics of the Arctic environment include various types of the permafrost, abundance of water, sharp changes in vegetation forms, snow and ice seasonalities, migrating animals (reindeer and birds), the high sensitivity of natural systems to climate change as well as the presence of relevant socio-economic-cultural aspects such as strong indigenous culture and livelihood, industrial development, a large non-indigenous population, and affluence of the region because of the natural resource extraction industries. These same features can be found in other regions of the Arctic such as Alaska, Arctic Canada and Fennoscandia. However, only in Yamal do they occur in such a high density, which makes this region exemplary and suitable for the development of convergence science frameworks.
The Yamal Peninsula, West Siberia, Russia represents a microcosm of the changing Arctic, where the two novel stressors described in Section 2 interact with a dynamic social ecological system. The peninsula is a clearly bounded natural laboratory with a biogeographic gradient from the forest-tundra ecotone to the high Arctic (Figures S1a–S1c in Supporting Information S1) and extends over 700 km south-north (240 km east-west) from the northern terminus of the Polar Urals to the Kara sea, presenting four of the five Arctic bioclimatic subzones, from subzones E, D, and C in the main land of the peninsula, to subzone B in the adjacent Belyi Island (Walker et al., 2005). Yamal is predominantly underlain by the continuous and in the south by discontinuous permafrost (Figure S1b in Supporting Information S1). Yamal evinces variation in both plant and animal communities along a latitudinal gradient. For vegetation, there is a general decrease in productivity, height and biodiversity of plant types; and there is an increase in the ratio of mosses to vascular plants from south to north (Walker et al., 2009). There is also a decreasing number of animal species from south to north (Ryzhanovsky et al., 2016). These plant and animal gradients mirror those seen elsewhere in the Arctic across bioclimatic subzones.
The region is a hotspot of surface air temperature warming: June–July warming over the period 1991–2020 has led to an increase of +1.32°C as compared to the climate normal period of 1961–1990, or +2.02°C, relative to preindustrial levels (1850–1900), far exceeding the range of natural climate variability (Hantemirov et al., 2022). Mean annual temperature changes from 1961 to 1990 to 1991–2020 are about +1.5°C (Malkova et al., 2022). There is a positive trend in liquid precipitation (mean total annual is 390 mm, 2000–2019), accompanied by a decrease in snowfall (mean total is 223 mm), and an increased likelihood of rain-on-snow events (Forbes et al., 2016). Rapid warming increases the vulnerability of the permafrost to thawing (Malkova et al., 2022; Shpolyanskaya et al., 2022). Borehole measurements indicate one of the fastest warming rates of ground temperatures across the Arctic regions with continuous permafrost (Biskaborn et al., 2019), although this can vary with the type of landscape (Kaverin et al., 2017). As in much of the Arctic, warming has been linked to an increase in height and abundance of tall shrubs and a shift of the forest-tundra ecotone in the southern half of the peninsula (Frost & Epstein, 2014; Hantemirov et al., 2008; Mazepa, 2005; Shiyatov et al., 2007).
Considerably less data have been published to date concerning responses of terrestrial fauna in Yamal to climate change. However, studies do suggest that animal species' ranges have shifted northward with climate change, leading to the “borealization” of small rodent and bird (Sokolov et al., 2012) communities, and expansion of breeding ranges of red foxes (Vulpes vulpes L.) and hooded crows (Corvus cornix L.) (Sokolov, Sokolov, & Dixon, 2016). These have important consequences for food web structure and functioning (Ims et al., 2013a, 2013b).
The built environment on Yamal includes towns and villages developed over the last 90 years, as well as a network of trading posts (faktoria), industrial extraction sites, and slaughterhouses. Linear infrastructures include a railway, and some short concrete roads. Long distance roads are maintained as ice-roads during the winter season. Industrial development has increased rapidly since the 1990s (Stammler, 2011). The largest industrial facilities situated in Yamal are Bovanenkovo, Sabetta, Noviy Port and Kharasavey, with several thousand workers each. The footprint of infrastructure and urban development in Yamal is not large in a spatial context, but much of the new infrastructure is linear and connects previously remote and relatively isolated communities (Kumpula et al., 2012). The 572 km Obskaya–Bovanenkovo railway (Figures S1b–S1c in Supporting Information S1), completed in 2011, is the world's northernmost railway (Terekhina & Volkovitskiy, 2020). Industrial activity brings large numbers of shift workers, commuting between urban centers in Yamal-Nenets Autonomous Okrug (YaNAO) and other cities of Russia and fly-in/fly-out settlements, such as Sabetta and Bovanenkovo. Industrial commuters, thus now outnumber the permanent population of native villages. Important for this paper are new stressors associated with industrialization, specifically the growing presence of industrial activities, such as construction in previously hard-to-access places, maintenance and transport of equipment and supplies for the gas industry, resulting in the continued development of infrastructure in the tundra. The challenge of complex, intertwined natural, social, and built environments on Yamal exemplifies why convergent science is necessary to tackle associated research questions.
Within this rapidly changing natural environment on Yamal is a complex social system including indigenous Nenets families living as nomadic reindeer herders and semi-nomadic fishermen, small majority-indigenous villages, and shift workers at infrastructure facilities. The number of permanent residents in Yamal is ca. 17,000 people, and almost 13,000 of them are indigenous peoples (mainly Nenets). The official number of shift workers is 25,000, but we assume that this data is underestimated (Loginov et al., 2020). Approximately 5,500 indigenous people engage in reindeer herding and fishing in the tundra, in a fully nomadic lifestyle with yearly migrations on reindeer sledges of up to 1,200 km (Terekhina & Volkovitsky, 2023). Yamal is one of the few places on the planet where people maintain the kind of nomadic pastoralism where moving is the norm rather than the exception. From the 1960s to present, the domestic reindeer population has grown from between 103,100 and 175,300 in 1990 (Makeev et al., 2014) to approximately 225,000 today (Terekhina & Volkovitsky, 2023). Previous studies (Forbes et al., 2009; Stammler, 2005) argued for strong resilience of reindeer herding lifeways within Yamal socio-ecological systems.
Today, independent households privately manage 80–90% of domestic reindeer in Yamal, while the rest of the herds belongs to a municipal enterprise (one former collective soviet “sovkhoz” that still remained in 2021). While the herding families spend most of their time migrating, most tundra people are registered in one of the villages of Yamalskiy district and children attend school there: Yar-Sale (the district center), Salemal, Syunai-Sale, Panayevsk, Novyi Port, Mys Kamenniy, and Seyakha (Figure S1c in Supporting Information S1). These villages contain core social institutions and infrastructure including administrators, schools, and clinics (Stammler, 2005; Ziker, 2002). Villagers maintain social and cultural relations with their nomadic relatives (Liarskaya, 2016; Volzhanina, 2017). Larger municipalities, such as Salekhard (the capital of YaNAO), have more complex social organization and infrastructure and indigenous leaders (natsional'naia intelligentsia) often reside there.
Beyond the growing development of infrastructure in the tundra, industrialization of Yamal has affected indigenous peoples living in Yamal in other profound ways such as through improved connectivity (expanded cellular network coverage (Stammler, 2009; Stammler, 2016), abundance of government sponsored satellite phones provided for the tundra families, transportation options and government sponsored train tickets) as well as expanded options for goods delivery, fuel supply, healthcare, veterinary services, and selling reindeer meat and fish to shift workers, etc.
Rapid climate change is increasingly impacting reindeer herders (Stammler & Ivanova, 2020). One type of event, rain-on-snow, leads to icing on pastures and inaccessibility of forage for reindeer in winter (Bartsch et al., 2010; Forbes et al., 2016; Sokolov, Sokolova, et al., 2016; Volkovitskiy & Terekhina, 2020). The most prominent of these entailed a loss of approximately 60,000 reindeer on the south Yamal Peninsula during the 2013–2014 winter. In 2018/2019, herds experienced local icings. In the winter of 2020–2021, up to 15,000 domestic and an unknown number of wild reindeer perished in northern Yamal, despite supplemental feed delivered to tundra by the regional authorities using specially chartered aircrafts. A convergent science approach lends itself to understanding the trade offs for various strategies that Nenets reindeer herders employ for dealing with these increasing climatic challenges.
3.1 Eco-Gradient
3.2 Rapid Climate Change
3.3 The Built Environment
3.4 Social System
4 Convergence Science Method
The research questions considered here are examples of multi-disciplinary convergence science questions, which resulted from a multi-stage brainstorming process undertaken by the author group. We pose it as an example of “engineering” an approach to facilitate effective and productive communications and generate a convergence science approach to research. This method, while not without its shortcomings, illustrates the value of an explicit structure for interactions within a heterogeneous expert group to yield integrative thinking—a prerequisite for the development of convergence science. We believe this method may be applicable to any group seeking to generate broad, important questions that rest on pillars of disciplinary knowledge. We describe it below, starting with an outline of our strategically structured workshop and “rules” of discussions that led to drafting convergence science questions, some of which are presented in this article.
In March of 2020, the rapidly evolving COVID-19 epidemic resulted in travel restrictions that disrupted plans of our team for an in-person meeting. We thus organized an online workshop. We set the goal of identifying high impact convergence science questions that spanned the breadth of disciplines represented by the group in social, natural, and built-environment systems. Specifically, we consisted of social scientists (4), engineers (4), earth system scientists (7), and ecologists (12) from across Russia (11), Europe (4), Middle East (1), and the US (11) and one logistical issue limiting our day-to-day interactions was that the team members were separated by as many as 11 time zones. Beyond discipline and space boundaries, we were scholars of various cultural identities and, described broadly, the team consisted of North Americans of European, Russian, and Asian descent, Western Europeans, Asians, and Russians. While the workshop team did not include non-scholar participants, several co-authors had conducted long-term studies (for more than a decade) in the communities of Yamal and developed broad social networks that included indigenous reindeer herders, public organizations of indigenous peoples of Yamal, leaders of reindeer herding communities as well as various Yamal government departments. These stakeholder groups therefore furnished information input for the exemplary research questions of this manuscript—as mediated by our co-author experts who provided the necessary competence for translating the knowledge and needs of the non-scholar stakeholders into a culturally appropriate discourse during the convergence science process. The topics encompassed climate change, reindeer herding management, burgeoning industrial development, and others.
Our key challenge was to find the grounds for science questions that emerge far beyond the disciplinary expertise of any single group member or subset of the larger group. This required systematic efforts that would push workshop discussions out of disciplinary silos and concentrate them on the development of views and questions in which no single expert group could claim dominant expertise. As a result, we structured the workshop to start within our disciplinary comfort zones, then to gradually integrate disciplines in a hierarchical manner, to culminate in a drawn diagram of connections between elements of the Arctic system from which we could distill high-impact convergence science questions (Figure 1). Our goal at each phase of the workshop was to identify critical elements within multiple disciplines (such as reindeer, arctic fox, herders, permafrost, shrubs, etc.), and then determine connections among these elements defined by explicit mechanisms—even if they remained elusive or unknown due to missing expertise in the team. The focus on elements connected by mechanisms kept all discussions grounded in practical rather than abstract terms. This facilitated discussions in which element-mechanism interconnections would be converted into concrete research objectives driven by testable science hypotheses and questions. The workshop participants also regulated discussions to prevent their swaying toward non-actionable science (i.e., too remote from team's expertise or too uncertain due to current inability to observe or measure relevant processes). This prevention emerged as a social norm during workshop discussions by the participants, rather than imposed as a “rule of the conduct” (which would have probably limited brainstorming and original thinking by individuals to some extent).
The diagram shows a hierarchical design for structuring a Workshop to discover convergence science questions that emerge from multi-discipline integration. The workshop began with disciplinary presentations (“EP”) grouped by science themes (“Theme”). Disciplines were integrated by identifying “linkages” defined as mechanistic connections between elements, first within Themes, then across Themes, and finally throughout a unified network model of the study system. Remote Breakout Groups were structured geographically to accommodate time-zone variation. Full Group Discussions at beginning, middle, and end of the workshop encouraged full scientific and cultural integration.
We began with recorded 15-min expert presentations (Figure 1, “EP” elements at the base of the workshop pyramid) by select members to present key disciplinary questions to non-experts of the discipline (i.e., representatives of other disciplines). Presentations were grouped into four “themes” combining similar fields: Theme I—“Arctic climate, weather, hydrology, permafrost, landscape vegetation,” Theme II—“Herbivory, predator-prey interactions, birds,” Theme III—“Plants, productivity, nutrients, dendrochronology, paleo-botany, ontology, ecology,” and Theme IV—“Social anthropology: human-environment interactions, reindeer herding in Yamal.” These expert summaries were foundational for building a collective language of communication among the participant scientists. Overall, we had 11 expert presentations within the context of the four Themes. Indeed, the act of summarizing key disciplinary questions in a language that non-experts could understand forced each expert to break down those questions to their most fundamental and important elements. This allowed each to step into the shoes of the other participants–to consider the question, “why is this important to others?” That in itself turned out to be an important key to our convergence science approach, one challenged to integrate ideas from vastly different disciplines.
The first challenge for each participant was to identify one mechanistic linkage between two elements of high scientific interest drawn from different presentations within each theme. By initially bridging related disciplines, these linkages formed the foundation for the gradual building of cross-disciplinary networks. Two such example linkages could be: shrubs (element 1) elevate winter soil temperature (element 2) by trapping snow (mechanism); linear infrastructure (element 1) increases fox abundance (element 2) via human-discarded food subsidies (mechanism). It was critical to keep linkages transparent and formulaic, avoiding abstraction or grouping of concepts. Thereby, when linkages were later connected into networks crossing disciplines, they could represent manageably sized research questions containing measurable elements and mechanisms.
Within the subsequent breakout groups corresponding to geographic regions of the team (Figure 1, “B” elements), each group discussed the individual linkage choices, and attempted to modify and distill two top linkages that crossed disciplines (within themes) and appeared to present opportunities for novel science. For example, while the effect of shrubs on snow retention has been well studied in the plant and biophysical sciences, the effect of shrub distributions (element 1) on ptarmigan population structure (element 2) via landscape snow redistribution (mechanism) is more likely approaching novel scientific territory by bridging related biological and biophysical disciplines. Most groups also opted to create thematic network diagrams to gain a more holistic understanding of the emerging science in preparation for later integration across themes. In a desire to avoid established questions of disciplinary interest and facilitate our forging into novel convergence science territory—in which no group member could claim expertise—we chose to have each breakout session led by a non-expert of the theme, while thematic experts were assigned rapporteur roles and were directed to express their opinions last.
Our next challenge was to consolidate the regional consensuses on linkages that were identified to have potentially novel science. We worked through two full-group meetings (Figure 1, “G” elements) to debate and edit the 12 linkages from two Themes identified by the breakout groups, and ultimately integrate them into two “spaghetti diagrams” (an example of one is in Figure 2a). This step required the identification of missing links, especially between science themes, thereby expanding the emerging convergence science pathways. During this integration process, the team also contextualized the developed spaghetti diagrams from a regional perspective: we explicitly considered the question of what makes Yamal a scientifically appealing place to study questions of interest (Figure S1 in Supporting Information S1). At this stage we also filtered out what was viewed as apparently non-actionable science (still, this was done in a conservative fashion to minimize a disregard for high-risk, high-yield research areas).
(a) A “spaghetti diagram” illustrating one outcome of group discussions (Figure 1, “G1” element) of Theme 1 and 2. (b) A “Unified Model” of Arctic elements and linkages achieved during the Great Spaghetti Cook-Off, the culmination of our convergence science workshop. Bubbles are “elements” of high interest identified and distilled throughout the workshop, colored by category (see Key). Arrows represent connections with explicit mechanisms identified during the workshop. After generating the fully integrated network model, three Breakout Groups each determined two science threads, each containing five linkages, depicted as colored connection-arrows. With each science thread, we aimed to represent integrated science questions of high impact that were plausibly testable and could not be addressed within any single discipline. The Unified Model and science threads are products of rapid brainstorming and iterated distillation, which formed a useful foundation for later work to formalize specific science questions and work plans.
After synthesizing pairs of Themes, we divided again into regional breakout groups tasked to “distill” the two spaghetti diagrams (Figure 1, “D” elements). Distillation entailed applying “Occam's razor” to diagrams overpopulated with elements, and highlighting key linkages leading to apparently novel convergence science (Figure 2a). We again made a concerted effort to avoid the tendency for abstraction, and maintain networks made of observable elements connected by explicitly hypothesized mechanisms. At this stage we began to formulate higher-level science questions that could be informed by an integrated study across a trans-disciplinary chain of elements.
Our final challenge via full-group discussions was to integrate the distilled spaghetti diagrams into a single unified network diagram and identify high-impact and actionable convergence science questions that arise from that network (Figure 2b)—a process that we referred to as “The Great Spaghetti Cook-Off” (Figure 1, the top of the workshop pyramid). Prior to this meeting, the distilled spaghetti diagrams were integrated into a single network containing all of the elements and their connecting arrows (as presented in group distillations), with elements color-coded by themes, ready for live editing during the full-group meeting. First, the full group debated and refined the network structure and labels. Then, we divided into three sub-groups, each of which was tasked over 45 min to develop two science Threads—a sub-network of five elements connected by mechanistic linkages within the integrated spaghetti diagram. Each Thread was to represent elements and processes of high scientific interest, with perceived strong mechanistic interactions between elements from different disciplines. We then reconvened as a full group to discuss and refine the Threads, ensure their mutual distinction, and critically re-evaluate the elements and mechanisms that had not been assigned to any Thread. Our final product was a cohesive network diagram with six color-coded Threads (Figure 2b). At the workshop closure, by tracing mechanistic pathways in each of the Threads, our team thus drafted tractable convergence-science questions of highest interest that were best informed by cohesive inputs from multiple disciplinary studies. We felt that these questions, by the nature of their construction through our workshop design, necessarily forged into novel scientific territory, successfully producing convergence science objectives.
Our convergence science questions were further refined in post-workshop activities led by team sub-groups, whose composition continued to represent diverse areas of expertise. For each Thread and its corresponding science question, the sub-groups were tasked to refine and detail hypothesized conceptual models of mechanistic pathways connecting Arctic stressors to their direct impacts. They were also called to consider effects exerted on elements of natural, social, and built-environment systems, as well as triggered responses and adaptive strategies. Three examples of such convergence science questions are provided in the next section.
Overall, the described method proved successful as a foundation for the convergence science questions presented here and for a subsequently funded grant proposal (through the National Science Foundation “Navigating the New Arctic” program). In addition, the group members found the process highly stimulating, enhancing cross-cultural and cross-disciplinary connectivity, broadening each of our knowledge bases, and improving understanding of how each of our research foci fits into a broader picture of Arctic processes.
5 Convergence Science Threads
During a workshop in 2020 and subsequent synthesis activities, the authors co-developed several convergence science questions whose causal mechanisms were perceived to be of high priority to understand. The objective for the selected three research threads below is to illustrate how a given question relating a certain stressor (i.e., climate change and industrialization) and an Arctic system agent(s) (e.g., reindeer, herders, tall shrubs) can be addressed via the development of a holistic view of system interactions and their spatial and temporal scales. Specifically, we illustrate how the integration of disciplinary knowledge of processes and the relevant linkages can lead to conceptual models of interactions in a network of interlinked elements. Such models can then serve to identify specific causal connections that can be addressed in research to further our understanding of overarching mechanisms. We also formulate Emerging Questions (denoted as EQ) that are important for the overarching research thread question and currently represent knowledge gaps.
Research question 1.How does expansion of increasing human presence and the built environment impact animals?
The growing presence of an indigenous population (with their reindeer) and newcomers (with their infrastructure) has complex impacts on local animal species (Figure 3). For example, food subsidies grow following an increased number of reindeer (Ehrich et al., 2017; Sokolov, Sokolova, et al., 2016). Additionally, more people in tundra potentially means an increase in food waste and anthropogenic food subsidies. On the other hand, domestic dogs can prevent endemic mammalian scavengers from accessing subsidies in settlements of hydrocarbon extraction fields.
A conceptual diagram of agents and effects (ovals) and processes (arrows) linking the built environment with ecosystem elements in Yamal: stressors (gray), direct impacts (yellow), and faunal responses (orange).
Infrastructure can reduce and fragment natural habitats, but at the same time can create new habitats for some species. This may benefit generalist predators, such as corvids and foxes and alter predator-prey relationships, potentially leading to an increased predation pressure on wild prey species, such as ground-nesting birds and rodents. This can also lead to a discussion of the context of arctic fox in relation to reindeer (Terekhina et al., 2021). Effects are further complicated by climate-related phenomena, such as prolonged winters (late springs) leading to delay in arrival of migratory birds, another important fox food source. This highlights the complexity of relationships between infrastructure and increased human presence on the one hand, and wildlife on the other.
The Obskaya-Bovanenkovo railroad (Figure S1b in Supporting Information S1) presents an example of how infrastructure development impacts wildlife on Yamal (Sokolov et al., 2017). The railroad extends from the forest tundra in the south to the high Arctic in the north and crosses numerous rivers. Bridges associated with the railroad have allowed the expansion of new species into the Arctic zone. Ravens and gyrfalcons did not breed in Yamal outside of forested areas and rocky cliffs prior to 2011, when the railroad was constructed. Bridges associated with the railroad provide elevated nesting sites previously unavailable to ravens. Gyrfalcons followed the ravens northward, utilized their nests, and flourished. This led to the first documented gyrfalcon breeding in Yamal, where the flat landscape does not provide sufficiently high natural rock cliffs or tall trees for this species to breed. Expansion of this top predator along the Yamal railroad potentially has an impact on prey populations too, especially ptarmigans. The raven population, which preys on nests of numerous birds, could likewise have a negative effect on avian species, such as grouse and waders (Henden et al., 2021; Rød-Eriksen et al., 2020).
EQ (Emerging Question): How does human activity modify top-down control of trophic interactions in tundra food webs? How does climate change impact the effects of anthropogenic disturbances on ecosystem dynamics?
Research question 2.How do warmer winters and seasonal shifts transform human and reindeer lives in the tundra?
Changing seasonality of winters affects both reindeer herding and systems that provide services to communities in the region. We hypothesize that warm spells throughout winter make mobility and transportation on Yamal more difficult. The start of the winter season with snow and ice determines the pace of reindeer herders' migration and camp movement to meat processing facilities where herders get their main yearly income. These migratory patterns, where timing is key, are such that some rivers need to be solidly frozen to be crossed with camps and herds. Research question 2 explores how warmer winters and seasonal shifts affect both reindeer herding and mechanized transportation (Figure 4).
A conceptual diagram of the mechanistic linkages between the stressor (gray), direct impacts (yellow), effects (orange), and adaptive strategies (green) taken by reindeer herders facing warming winters. The arrows represent the direct and bidirectional effects of the linkages.
Unpredictable winter seasonality results in bottlenecks for larger households during autumn and spring Nenets migration schedules. These delays create herbivory pressure along transit routes on both sides of the major water bodies, where nomads are “stuck” waiting for the ice. This, in turn, can lead to conflicts. Smaller private herders that rely on these pastures for their winter grazing close to villages (Yar-Sale, Ports-Yakha, Salemal, Panaevsk) complain that big herds that are supposed to go to the other side of the wide Ob’ River destroy their winter grazing areas. Now, more Nenets aim to leave the peninsula for winter, as they are not satisfied with tundra pastures during winter. This leads to a higher concentration of herds waiting on the banks to cross rivers, resulting in high pressure on pastures. EQ: What is the role of concentrated urine and defecation resulting from concentration of herds? What are the resulting changes in biomass of plant communities and how are they altered, potentially reducing, or improving overall pasture quality?
On the other hand, herders report that some of this grazing pressure will be “taken back by nature” (Russian: priroda voz'met svoyo), when large iced-covered patches of pastures caused by rain-on-snow (ROS) events become inaccessible for longer periods. EQ: To what extent does ROS allow the pastures in those areas to recover, by reducing the density of animals grazing and moderating the negative impact of trampling?
The most obvious disturbance for herding migration patterns and danger for nomadism as a livelihood as well as for herding as an economy is the impact of the increased frequency of ROS events and thaws (Serreze et al., 2021; Stammler & Ivanova, 2020). Recent publications have already shown these tremendous impacts (Forbes et al., 2016), and how herders respond to them (Golovnev, 2017; Stammler & Ivanova, 2020). These events do not necessarily increase competition among herders for scarce resources. A smart Nenets strategy relies on the animals' autonomous survival skills in the times of crises (Stépanoff et al., 2017). In Yamal, this is known as free grazing, where herders release their herds to roam freely, refrain from any herd control, and hope that the animals find pastures somewhere, even if it results in mixing with other herds. When a herd moves on their own, the owners can avoid direct confrontation with other herders by arguing that the herds are not driven by the people on purpose. EQ: Given increasing controversy about supplemental feeding in Fennoscandia (Horstkotte et al., 2021; Pekkarinen et al., 2021), how might a switch to intensive supplemental feeding change dependencies on state subsidies and affect reindeer health on Yamal? Would supplemental feed help vegetation recovery or reduce reindeer mortality? What combination of meteorological events leads to an ice crust critical for winter reindeer grazing?
Early winter road melting in spring means the danger of winter roads collapsing under big trucks that carry heavy loads. In extreme years, some winter roads do not open. For example, in 2019–20, the winter road from Salekhard, the district center, to Yar-Sale, the administrative center of the Yamal Peninsula, remained closed leading to increasing prices of all goods in the village. Reindeer herders responded to these pressures by purchasing more in Nadym, a town in the forest zone in the area of winter campsites and transporting goods on snowmobiles to the Peninsula. Some even took it as a business opportunity and hauled barrels of petrol from Nadym for sale in Yar-Sale. Not every family has the opportunity for such plastic responses to weather.
EQ: What is the effect of such events on equality of access to mobility and goods for the residents of remote villages lacking the formally established communications? How much warming will it take to cause a shift in the kind of transportation that is needed in winter along the Ob’ River?
Research question 3.How does summer heat affect reindeer herding?
Increased heat content in the atmosphere during snow-free summers in particular has significant impacts on reindeer due to the higher rate of biotic and human activities during this season (Figure 5). We hypothesize that the increased summer heat alters reindeer migratory patterns via impacts on landscape fragmentation by novel processes (shrubification, permafrost wasting, fire, and shifts in vegetation composition) that affect foraging, disease, insect harassment, and mobility. We additionally hypothesize that landscape constraints interact with socially negotiated access to migration routes of herding groups, leading to reduced flexibility in adaptation choices.
A conceptual diagram of the mechanistic linkages between the stressor (gray), direct impacts and process amplification (yellow), effects (orange), and adaptive strategies and reindeer health impacts (green) due to increase in summer heat. The arrows represent the direct and bidirectional effects of the linkages.
The direct implications of weather-scale change in the atmospheric heat content are well-characterized (Figure 5, “Reindeer heat stress”): reindeer have poor tolerance for high ambient temperatures and they avoid overheating through reduced metabolism and foraging only during night hours (Klokov & Mikhailov, 2019), often leading to animal weight loss. Furthermore, according to the Nenets, extreme summer heat leads to calves' lung disease (pers. comm.). Less apparent effects of heatwaves are due to the acceleration of frozen ground thaw (Figure 5, “Soil thaw; wet substrate”), which may create conditions of foraging in high wetness conditions. In combination with high temperatures and reduced mobility, this may force the herd to stay in trampled, boggy grazing pastures, that is, favorable conditions for hoof bacterial infections via scratches or wounds (Riseth et al., 2020). Heat waves are also associated with weather patterns during which low, calm winds can promote animal stress due to mosquito, warble flies, and nose bot flies harassment that inhibit reindeer foraging (“Low wind conditions”). Hagemoen and Reimers (2002) argued that the resultant decrease in feeding and resting and increase in demanding activities may compromise reindeer physical fitness, with possible consequences for winter survival. Excess summer heat therefore affects reindeer and nomads by reducing their mobility, with at least three negative effects: inhibiting efficient foraging to develop fat stores needed for winter survival (Nilssen et al., 1984); by increasing the spread of pathogens among dense, stationary reindeer; and by reducing the survival rate of calves in winter.
EQ: How will the continued increase in summer heat affect reindeer health, body conditions and their winter survival? Is there a particular migration or pasturing pattern that is more conducive to hoof infections (e.g., a rapid south-north migration on partially thawed surface vs. summer pasturing phase on warmer but wet soil)? Can these local, landscape-niche level effects of thaw feedback onto nomad migration patterns?
Furthermore, hot weather increases flammability of vegetation, making tundra (summer pastures) and boreal forest (winter pastures) more susceptible to ignition (Figure 5, “Tundra and forest fire”). Tundra fire has been observed to increase in the Arctic (Witze, 2020), likely due to the combination of lightning activity and frequent dry tundra conditions (He et al., 2022). Studies in Siberia are infrequent but indicate massive fires in the forest areas of mixed genesis, citing anthropogenic impacts (Moskovchenko et al., 2020). Fires can increase ecosystem productivity in tundra ecosystems, and may promote biodiversity (Heim et al., 2022) and active recruitment of shrub species (Myers-Smith et al., 2011) that can partly offset the fire depletion of biomass. However, fires also lead to the loss of slowly growing, energy-rich lichen—the preferred, if not dominant, winter nutritional element of reindeer (Turunen et al., 2009), highlighting specifically the vulnerability of winter reindeer pastures.
EQ: Can the loss of lichen due to the changing fire regime in the forest and tundra place additional pressures on the mobility of nomads and reindeer and social negotiations among herders, during both summer and winter periods? Can fire patchiness in the landscape impose violations of traditional reindeer foraging boundaries? Can the patchiness of fires impact the size of grazing herds as larger herds need larger pastures not impacted by fires?
Wind is one of the key determinants of mosquito relief (Skarin et al., 2004) and heat stress relief and higher coastal winds in Yamal are a strong enough feature of attraction for the migrating nomads. Coastal areas also have herbaceous plants of higher nutritious content that are forage for the reindeer. EQ: Will productivity of forbs in northern coastal areas increase with summer warming?
The effects of long-term, climate-scale changes in the atmospheric heat content are less understood, as they may initiate and convey processes that evolve over similarly long time scales (Ims, Jepsen, et al., 2013). Warmer summers, a deepening of the soil active layer due to the increased permafrost thaw, and the lengthening of the phenological period of photosynthesis have facilitated the growth of tall, woody shrub vegetation in tundra, though the main areas of growth cluster around water tracks and more severe active layer erosion (Elmendorf et al., 2012; Myers-Smith et al., 2011) (Figure 5, “Tall shrub expansion”). Studies in Fennoscandia showed that reindeer foraging can hold back shrubification of tundra (Horstkotte et al., 2017) but once sufficiently mature, shrubs may create localized impacts on herding practices. Herders typically avoid guiding reindeer through tall shrub thickets, so as to avoid reindeer feet and hoof injuries and excessive insect harassment, but they may also choose winter campsites in proximity to these thickets, which are a source of firewood and auxiliary subsistence material.
EQ: Can choices of pasture areas feed back onto the regional-scale vegetation cover by limiting shrub spread or mediating their distribution, for example, due to increased animal nitrogen enrichment via excreta? Overall, will the direct impacts of high ambient temperatures on reindeer and the indirect implications stemming from heat-induced changes in landscape lead to shifts in nomadic reindeer herding practices?
6 Discussion and Conclusions
The objective of this paper is to illustrate how convergence science—an approach that has seen increasing in interest over the past decade across a spectrum of disciplines (Thompson et al., 2023)—can be applied to the terrestrial Arctic system. While there has been general understanding that a convergence science approach bringing together earth systems scientists, social scientists, ecologists, and the affected stakeholders is needed, and science funding bodies over the world have recognized this and committed enormous resources to it (e.g., the US National Science Foundation's Navigating the New Arctic initiative), there are shockingly few publications on how to “do” convergence science, and none focused on studying the terrestrial Arctic system.
This paper presents a framework for breaking down disciplinary silos in bringing together social scientists, natural scientists, and engineers, and integrating the knowledge of non-scholar stakeholders. Our team, representing vastly different disciplines, countries, and cultural identities, came together to focus attention on climate change and industrialization and their impacts on the Yamal Peninsula of Arctic Siberia. This paper relays our process of design of convergence science questions and lessons learned during a hierarchically designed workshop in March 2020 that began with disciplinary perceptions of importance of Arctic system elements, and progressively integrated these views via specifically structured discussions into a unified network model. The latter served as a basis for the development of several convergence science questions, three of which were used as examples in this paper, aiming to showcase the identification of linkages critical to generating system-level understandings of stressor impact propagation. Overall, the discussed approach provides a foundation of value to a very wide audience—not just researchers and stakeholders interested in Arctic climate change or even climate change in general, but many interested in the convergence science thinking.
The exemplified convergent science approach has identified research questions that are broad in nature. As illustrated in their discussions, they do not have direct and immediate answers and cannot be fully addressed within a single disciplinary study because of numerous linkages conveyed as feed-forward connections between stressors, processes, and effects, and feedback mechanisms that are expressed as adaptive adjustments or strategies exhibited by Arctic elements. Nonetheless, because these questions were the result of integration, they can also be “differentiated,” that is, parsed into material links and tangible inquiries of disciplinary and interdisciplinary nature. While we present Yamal as a case-study region, many of the elements and their mechanistic connections presented here are broadly representative of the Arctic, and we believe our methodology for the discovery of convergence science can be applied to integrate disciplines in other systems of high complexity.
Even though not demonstrated in this paper explicitly, the process of mapping tangible questions onto a space of practical implementation is the next vital stage of the convergent science process. The illustrated development of question formulation is already tremendously useful for setting priorities among study elements and contemplating about the issues of research feasibility. But it is in the implementation stage that the research team may appreciate their gaps in expertise, methodology, and instrumentation. The team may further feel compelled to formulate additional—what we call here “emerging questions” (EQs), which can enrich and expand the scope of the overarching research thread. EQs are current knowledge gaps and stimulate a recursive (and iterative) assessment of thread linkages and content, particularly as new data and analyses start coming in to provide novel insights. It is also at that stage of the convergence science process that the relative importance of thread elements and their interactions and relevant mechanisms are re-assessed and convergence science questions are further scrutinized. Overall, a traversal of the entire convergent science pathway may characterize it not as an ultimately terminal endeavor, but as a learning process with ever-expanding dimensions of scientific inquiry.
Acknowledgments
V. Ivanov, P. Ungar, A. Sheshukov, D. Liu, and J. Wang acknowledge the support by the National Science Foundation (NSF) Navigating the New Arctic Program Track-II team planning Grant 1928014, 1927793, 1928020, 1928040, 1928061 and Track-I Grant 2126792 (Ivanov), 2126796 (Ungar), 2126794 (Ziker), 2126793 (Sheshukov), 2126797 (Wang), 2126798 (Liu), and 2126795 (Heskel). Additionally, Ivanov, Wang, Sheshukov, and Liu acknowledge the Office of Polar Programs (OPP) Grant 1725654, 1724633, 1724633, and 1724868. A. Sokolov, N. Sokolova, O. Pokrovskaya, P. Orekhov, A. Terekhina, A. Volkovitskiy, S. Abdulmanova, and I. Fufachev (all co-authors from Yamal) were supported by the Ministry of Science and Higher Education of the Russian Federation program, Grant 122021000089-9. V. Valdayskikh was supported by the state task of the Ministry of Science and Higher Education of the Russian Federation, project no. FEUZ 2024-0011.