Extramural Papers of the Month
December 2023: Extramural Papers of the Month Environmental Factor Newsletter
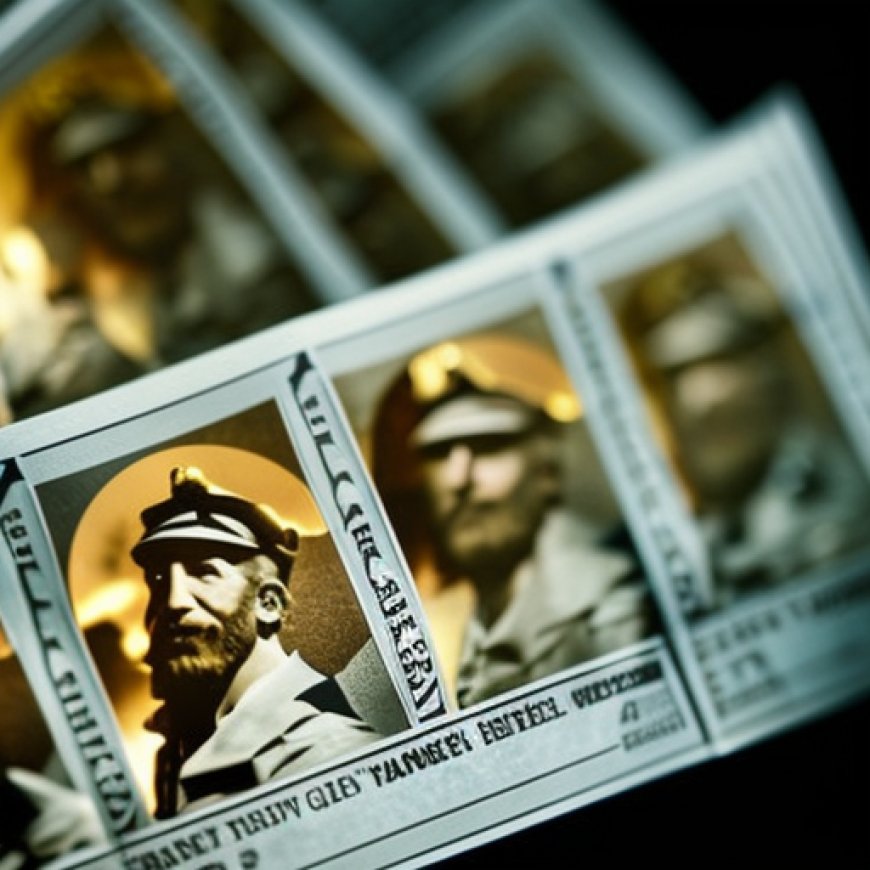
New method generates airborne free radicals for laboratory study
NIEHS-funded researchers developed a new approach to improve how environmentally persistent free radicals (EPFRs) are generated and studied in the lab. This strategy addresses a deficiency in methods to simulate realistic environmental exposures in animals.
Thermal remediation — a common approach for treating organic waste — can generate particulate matter containing EPFRs that vary in chemical composition based on their source. These compounds are highly reactive and can cause oxidative stress when inhaled. However, little is known about the human health effects of EPFR exposure, in part because relevant animal models and exposure techniques are lacking.
Scientists created and validated a novel combustion device to generate environmentally realistic EPFRs. They generated two batches of EPFRs that shared similar properties and a tendency to embed in the lungs when inhaled but contained either high or low radical concentrations. Team members also developed methods to aerosolize EPFR particles in the lab, more closely mimicking exposure conditions relevant to humans.
They compared mice exposed to filtered air with those exposed to air containing one of the groups of EPFRs. Mice exposed to EPFRs with higher free radicals had worse lung function and increased markers of vascular dysfunction. Those mice also showed greater activation of aryl hydrocarbon receptors — which play an important role in detecting and responding to pollutants — and increased expression of genes involved in antioxidant response.
The new method can produce more consistent particles and realistic conditions for EPFR studies, according to the team. It can also enable additional research investigating whether different combustion sources alter the mechanisms contributing to EPFR toxicity.
Citation:
Aryal A, Noël A, Khachatryan L, Cormier SA, Chowdhury PH, Penn A, Dugas TR, Harmon AC. 2023. Environmentally persistent free radicals: Methods for combustion generation, whole-body inhalation and assessing cardiopulmonary consequences. Environ Pollut 334:122183.
Microbial process for PFAS breakdown uncovered
A study funded by NIEHS revealed important mechanistic information about how some microbes break down PFAS in the environment. The findings may inspire more cost-effective bioremediation approaches.
The team focused on chlorinated PFAS (Cl-PFAS), which are increasingly prevalent and not well studied. Cl-PFAS have the characteristic strong carbon-fluorine bonds that are challenging to break, as well as weaker carbon-chlorine bonds, which may be more vulnerable to microbial breakdown — a process called dechlorination.
The researchers evaluated the ability of an activated sludge microbial community to break down 12 different Cl-PFAS compounds with varying structures and numbers of chlorine-fluorine bonds. Then, they measured the amount of the original compound and fluoride left in the samples. They also used nontargeted analysis to identify breakdown products that would indicate the mechanistic pathways by which microbes destroyed PFAS. Finally, they identified which microbes were responsible for PFAS degradation, and the relationships between their activity and Cl-PFAS structure.
The scientists reported a novel pathway used by the microbes to ultimately break the carbon-fluorine bonds, called defluorination, and degrade PFAS by first attacking the weaker carbon-chlorine bonds. The microbes dechlorinated Cl-PFAS compounds using one of three different mechanisms. The most effective, called hydrolytic dechlorination, led to the highest spontaneous levels of subsequent defluorination. Hydrolytic dechlorination relies on water molecules to break the carbon-chlorine bond. Cl-PFAS compounds with more chlorine atoms underwent more hydrolytic dechlorination, and therefore, defluorination. Genetic analysis of the microbial community showed that two bacteria, Desulfovibrio aminophilus and Sporomusa sphaeroides, dominated PFAS breakdown.
According to the authors, the findings can help inform the design of readily biodegradable alternative PFAS compounds, as well as more cost-effective bioremediation strategies.
Citation:
Jin B, Liu H, Che S, Gao J, Yu Y, Liu J, Men Y. 2023. Substantial defluorination of polychlorofluorocarboxylic acids triggered by anaerobic microbial hydrolytic dechlorination. Nature Water 1:451–461.
Higher summer humidity may increase hospitalizations for cardiovascular disease
NIEHS-funded researchers reported a link between exposure to higher humidity in the summer and more hospitalizations related to cardiovascular disease. Most climate-health studies focus on temperature, but increased humidity may be an important climate factor affecting health.
Because humid air limits sweat evaporation from the body, straining the cardiovascular system, the team set out to evaluate associations of long-term exposure to summer humidity and various cardiovascular system diseases.
The researchers used publicly available information for approximately 63 million Medicare beneficiaries living in the U.S. between 2000 and 2016. They assessed zip code-level summer average humidity and humidity variability based on daily estimates from the Gridded Surface Meteorological dataset. Using statistical models adjusted for individual and area socioeconomic status indicators, temperature, and winter humidity, they estimated associations between summer humidity and hospitalizations for cardiovascular disease, coronary heart disease, and cerebrovascular disease.
Higher summer average humidity and humidity variability were associated with an increased risk of hospitalization for all three diseases. These associations were more pronounced among those eligible for Medicaid, an indicator of low socioeconomic status, and with beneficiaries of unknown race or a race listed as “other.” Associations were not affected by adjusting for temperature or regions of the U.S.
According to the team, increasing humidity levels may be an important contributor to climate-related health effects.
Citation:
Klompmaker JO, Laden F, James P, Benjamin Sabath M, Wu X, Dominici F, Zanobetti A, Hart JE. 2023. Long-term exposure to summer specific humidity and cardiovascular disease hospitalizations in the US Medicare population. Environ Int 179:108182.
Multiple screening assays provide complementary data on potential chemical hazards
Combining information from assays using zebrafish and cells can quickly provide a more complete picture of potential chemical toxicity, according to a study funded by
SDGs, Targets, and Indicators
1. Which SDGs are addressed or connected to the issues highlighted in the article?
- SDG 3: Good Health and Well-being
- SDG 6: Clean Water and Sanitation
- SDG 7: Affordable and Clean Energy
- SDG 11: Sustainable Cities and Communities
- SDG 13: Climate Action
- SDG 15: Life on Land
2. What specific targets under those SDGs can be identified based on the article’s content?
- SDG 3.9: By 2030, substantially reduce the number of deaths and illnesses from hazardous chemicals and air, water, and soil pollution and contamination.
- SDG 6.3: By 2030, improve water quality by reducing pollution, eliminating dumping and minimizing release of hazardous chemicals and materials, halving the proportion of untreated wastewater, and increasing recycling and safe reuse globally.
- SDG 7.2: By 2030, increase substantially the share of renewable energy in the global energy mix.
- SDG 11.6: By 2030, reduce the adverse per capita environmental impact of cities, including by paying special attention to air quality and municipal and other waste management.
- SDG 13.2: Integrate climate change measures into national policies, strategies, and planning.
- SDG 15.9: By 2020, integrate ecosystem and biodiversity values into national and local planning, development processes, poverty reduction strategies, and accounts.
3. Are there any indicators mentioned or implied in the article that can be used to measure progress towards the identified targets?
- Indicator: Number of deaths and illnesses from hazardous chemicals and air, water, and soil pollution and contamination.
- Indicator: Water quality improvement through reduction of pollution and release of hazardous chemicals.
- Indicator: Share of renewable energy in the global energy mix.
- Indicator: Adverse per capita environmental impact of cities, including air quality and waste management.
- Indicator: Integration of climate change measures into national policies, strategies, and planning.
- Indicator: Integration of ecosystem and biodiversity values into national and local planning, development processes, poverty reduction strategies, and accounts.
Table: SDGs, Targets, and Indicators
SDGs | Targets | Indicators |
---|---|---|
SDG 3: Good Health and Well-being | Target 3.9: By 2030, substantially reduce the number of deaths and illnesses from hazardous chemicals and air, water, and soil pollution and contamination. | Number of deaths and illnesses from hazardous chemicals and air, water, and soil pollution and contamination. |
SDG 6: Clean Water and Sanitation | Target 6.3: By 2030, improve water quality by reducing pollution, eliminating dumping and minimizing release of hazardous chemicals and materials, halving the proportion of untreated wastewater, and increasing recycling and safe reuse globally. | Water quality improvement through reduction of pollution and release of hazardous chemicals. |
SDG 7: Affordable and Clean Energy | Target 7.2: By 2030, increase substantially the share of renewable energy in the global energy mix. | Share of renewable energy in the global energy mix. |
SDG 11: Sustainable Cities and Communities | Target 11.6: By 2030, reduce the adverse per capita environmental impact of cities, including by paying special attention to air quality and municipal and other waste management. | Adverse per capita environmental impact of cities, including air quality and waste management. |
SDG 13: Climate Action | Target 13.2: Integrate climate change measures into national policies, strategies, and planning. | Integration of climate change measures into national policies, strategies, and planning. |
SDG 15: Life on Land | Target 15.9: By 2020, integrate ecosystem and biodiversity values into national and local planning, development processes, poverty reduction strategies, and accounts. | Integration of ecosystem and biodiversity values into national and local planning, development processes, poverty reduction strategies, and accounts. |
Behold! This splendid article springs forth from the wellspring of knowledge, shaped by a wondrous proprietary AI technology that delved into a vast ocean of data, illuminating the path towards the Sustainable Development Goals. Remember that all rights are reserved by SDG Investors LLC, empowering us to champion progress together.
Source: factor.niehs.nih.gov
Join us, as fellow seekers of change, on a transformative journey at https://sdgtalks.ai/welcome, where you can become a member and actively contribute to shaping a brighter future.